Environmental monitoring using inexpensive microsensors is becoming increasingly prevalent and normalized in our daily lives. These sensors can tell us the temperature, humidity, and pollen count. They can detect the presence of a person at our door, or show us if a groundhog is eating vegetables from our garden.
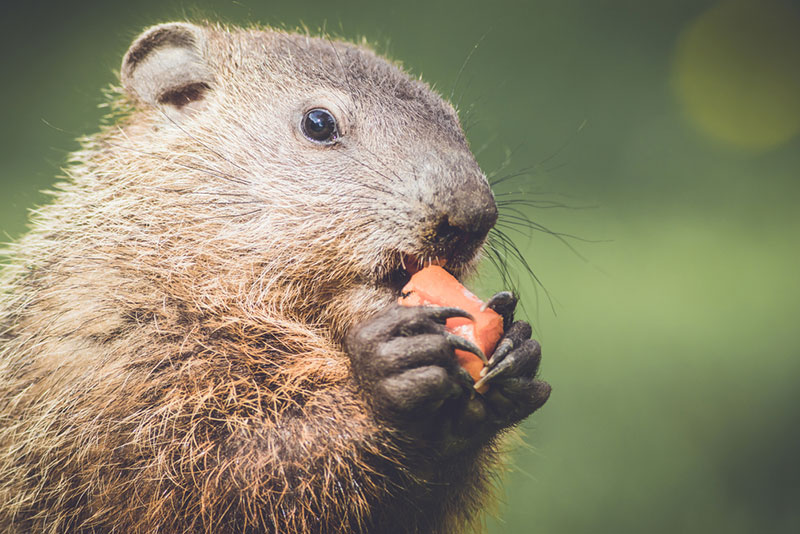
Even more intimate than monitoring our homes and gardens, people themselves are wearing sensors to track their heart rates, steps, calories burned, sleep patterns, and movements across the landscape. The thirst to digitize the world by adding more sensors, along with the ability to store the data they generate on huge data farms, is seemingly unquenchable.
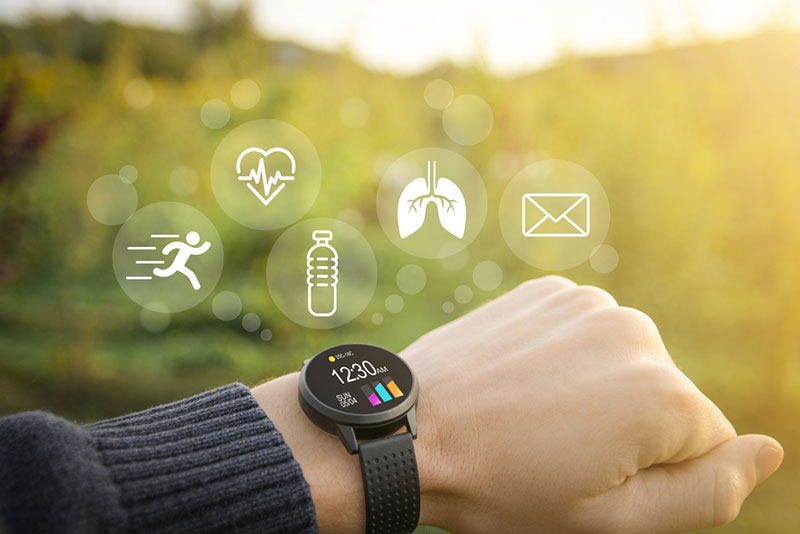
The rationale for collecting so much data may in part be due to an over-exuberance for technology, a “because we can” mentality, without a specific purpose. However, it’s also true that the growing network of sensors, along with cloud computing and controls, the so-called “Internet of Things” (IoT), is yielding important information that can be used not only to monitor conditions but also to provide feedback that helps us optimize performance and attain desired outcomes. Can sensors and the information they produce be used to optimize ecological functions? The arc of history from observation to science to technology suggests that humanity will try.
The use of sensors to monitor specific environmental parameters has been a tool in the field of ecology for decades. What’s notable are the advances that have been made in the past 30 years. Separate handheld probes to measure salinity, dissolved oxygen, and conductivity were merged into multimeters for grab sampling. The scientist once had to document the observations regularly in a field notebook, and usually do at least three readings to get an average to round out the variance in the probe’s display. Then, continuous data collection from sensors came onto the market.
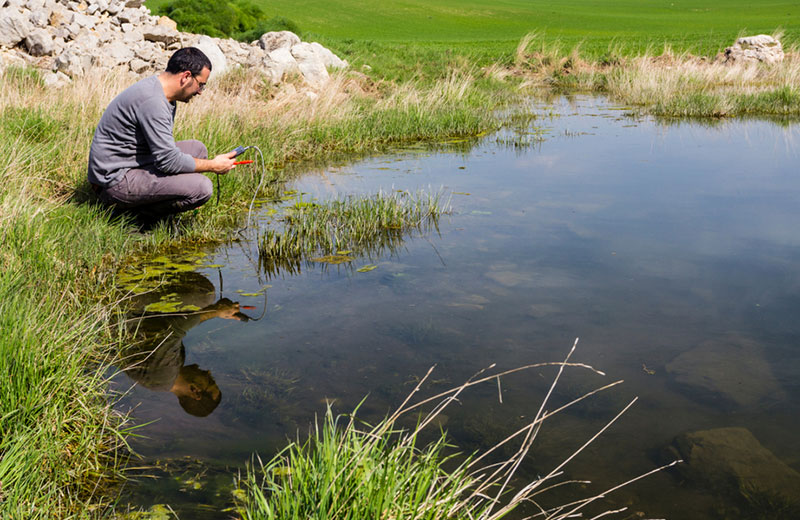
Scientist measuring environmental water quality in a wetland using a multi-parameter probe
Using rain gauges, pressure transducers, and temperature loggers that could monitor rainfall, water stage, and thermal patterns in streams and wetlands at specified intervals became a standard approach to evaluating the health of a stream and its watershed around the turn of the century. These devices store the data but require frequent downloading, requiring periodic field visits. More expensive sensing technology began using telemetry to transmit data to a server, which could then be shared in real time and inform management decisions. However, this technology has typically been applied to infrastructure projects rather than ecosystems. With the cost of sensors dropping and with the availability of wireless transmission of data and cloud storage, the technology is now accessible for homeowners to set up their own weather stations and check in on their gardens from their phones—or for environmental managers to test their assumptions about high-performance landscapes.
At Biohabitats, revering wild nature is a core value, so one might not expect us to actively explore leveraging the information gleaned from sensors to automate the management of an ecosystem. But in urban environments, where nature has been entirely supplanted by the built environment, we can’t expect to restore a self-maintaining and self-regulating wildness. We can, however, invest in supporting and improving the ecosystems we do have, to maintain biodiversity and enhance ecosystem services wherever possible and sensors can help us.
What seems clear is that the field of ecology is no longer a field of study that only observes, theorizes, analyzes, and reports on species and natural systems that are in decline. It must also offer evidence of whether (or not) conservation, restoration, and adaptive management practices are yielding positive ecological returns. Ecologists should use data from natural systems to steer us back on track to regenerating our living planet. The emergence of inexpensive environmental sensors, cloud computing, and controls represents a frontier in applied ecology; whereby cybernetic ecosystems can optimize the throughput of energy and matter to maximize the yield of ecosystem services. In some instances, giving ecosystems additional information and agency to control their throughputs can help protect the systems from increasingly frequent extreme events associated with climate change and large-scale landscape disturbances.
In Baltimore, as part of the Healthy Harbor Initiative which sought to make the Inner Harbor swimmable and Fishable (a goal that after 13 years has very nearly been realized), we looked for every opportunity to implement interventions that would increase awareness, improve water quality, and provide habitat and refugia to prevent fish kills. One such idea involved reclaiming and transforming the derelict Chase Pier into a “wet wharf,” a waterfront feature that would simultaneously serve as cultural artifact, habitat, water filter, and sentinel to engage the public in enjoying the Harbor and learning about its health.
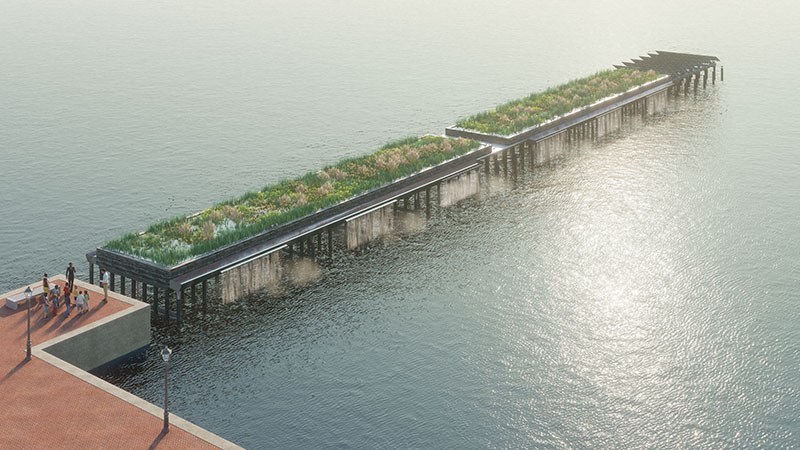
A reimagined Chase Pier
In addition to building a constructed, tidal marsh on the top of the pier, the design proposed a series of waterfalls cascading around its perimeter to combat the Harbor’s low dissolved oxygen. Given the episodic nature of low dissolved oxygen events, the waterfalls were designed to activate only when sensors detected perilously low oxygen levels. In this manner, the waterfalls assumed the role of a visual indicator for poor water quality while simultaneously providing a refuge, or “life zone,” for aquatic organisms during these life-threatening occurrences.
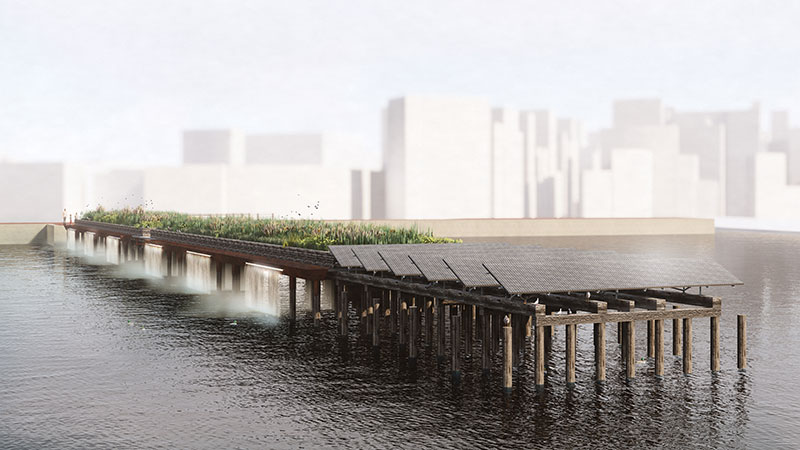
The Chase Pier project was never built, but IoT is automating management for enhanced ecosystem services in an urban environment at the Georgia Institute of Technology campus in Atlanta. Georgia Tech wants to reduce the annual volume of stormwater runoff from the campus by 50%, and they are looking to a campus space known as the EcoCommons to help. Located at the bottom of two small, urbanized catchment basins, the EcoCommons is intended to serve as a sponge and evapotranspiration machine for the drainage area above it. To function like a sponge, the 7-acre site is designed to absorb rain that falls directly on it. In addition, runoff from surrounding impervious surfaces is detained in underground cisterns and applied to the EcoCommons to create a mesic Piedmont forest condition. Soil moisture sensors installed throughout the landscape keep continuous tabs on the availability of water for plants. If soil moisture drops below a specified percentage, the harvested rain will be called to irrigate. As the plants mature into a Piedmont Forest, increasing volumes of water will evaporate into the atmosphere, helping to cool the site and temper the urban heat island. Finally, as the roots grow deeper and the soils become more porous, it is hypothesized that infiltration will improve with time.
These examples illustrate the advent of IoT that informs the management and function of a built ecosystem. Next Nature, a network of makers, thinkers, and educators, argues that biology and technology are fusing. In their Pyramid of Technology, Chase Pier was only envisioned, while the EcoCommons reached the applied step. In urban landscapes and waterfronts, how long will it be before the application of sensors, automated controls, drones, and robots become invisible, or perhaps nature? Maybe we’ll find the answer when groundhogs stop mugging for the camera, or when great white sharks trolling off Cape Cod demand their privacy.
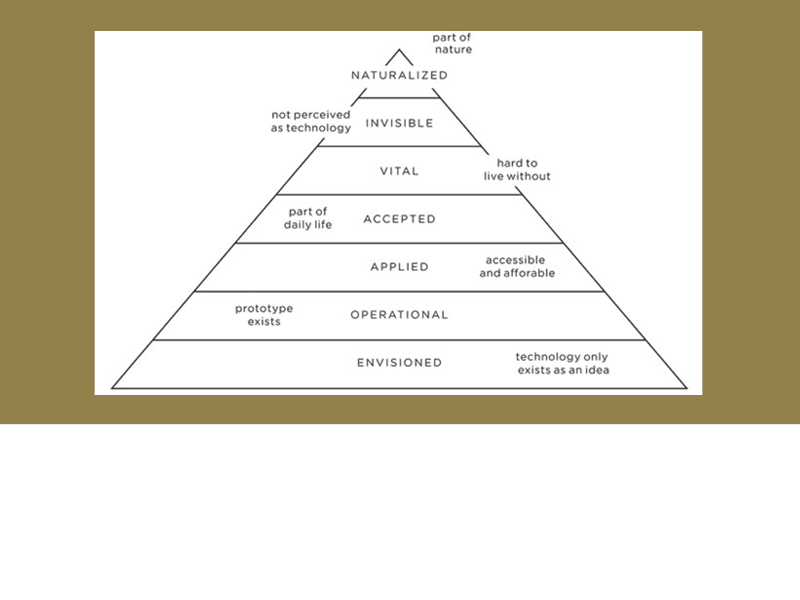
©Next Nature